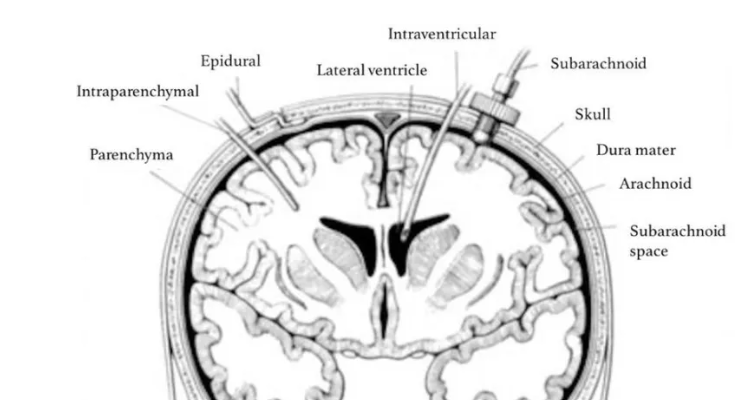
Article topic: ICP Monitoring: Types and Indications
Authors: Diana Bashashi, Wassim Hamadeh
Editors: Rahmeh Adel
Reviewer: Ethar Hazaimeh
Keywords: Intracranial pressure, cerebrospinal fluid, invasive monitoring, non-invasive, infection, hemorrhage, micro-transducer, optic nerve sheath diameter.
Abstract
Intracranial pressure (ICP) is the pressure within the craniospinal compartment, which comprises a fixed volume of neural tissue, blood, and cerebrospinal fluid (CSF). The importance of keeping this parameter constant and the signs and symptoms that should raise suspicion for an elevated ICP are described in this article.
This article aims to demonstrate the importance of using ICP monitoring systems, their types, and their indications in the management of patients with abnormal intracranial pressure due to intracranial pathologies or other medical conditions.
ICP monitoring is a powerful tool because an elevated ICP could lead to devastating neurological problems and ultimately to coma and death. Additionally, it has the potential to help physicians in their judgment of the correct timing of intervention to evaluate the therapy efficacy and as a consequence, to influence the clinical outcome in the short and long term.
Introduction
Intracranial pressure (ICP) is defined as the pressure exerted by the intracranial contents (blood, CSF, and brain parenchyma) on the dural envelope. The pressure inside the cranial vault is usually measured in millimeters of mercury (mmHg) and is under normal conditions lower than 20 mm Hg.
An abnormally high ICP may occur in a myriad of medical conditions, for instance, by any intracranial masses, either intrinsic to the brain parenchyma (e.g. a tumor like a glioma or a brain abscess) or extrinsic to it (e.g. an extradural hematoma). A diffuse brain swelling following severe hypoxia, ischemia, or Reye syndrome, in liver failure (uremic encephalopathy and hyperammonemia), hypertensive encephalopathy. It may occur following an impaired circulation, absorption, or production of CSF, such as in obstructive hydrocephalus, meningitis, and choroid plexus tumor, which might lead to CSF build-up around the brain. ICP might also get abnormally high in the context of skull deformities (e.g. craniosynostosis), trauma, and hyponatremia.
The skull and vertebral canal, together with the dura, form a semi-rigid box, such that any increase in the volume of any of its contents (neuronal tissue, blood, or CSF) will raise the ICP. These components exist in a state volume equilibrium so an increase in any one of these elements must be at the expense of the other two, otherwise, an increase of ICP will ensue (this is known as the Monroe-Kellie model and was stated centuries ago). In other terms, the combined volume of the intracranial content is constant under normal conditions and to maintain a homeostatic intracranial pressure, any increase or decrease in one of these elements, blood, CSF, or brain will lead to a reciprocal and opposite change in the others. However, because of the buffering effect of CSF being displaced from the cranial cavity into the spinal canal, minor increases in brain volume do not instantly induce an increase in ICP.
Once these compensating measures have been exhausted, any further augmentation in brain volume necessarily reduces the intracranial blood volume contained in the veins and dural sinuses which might jeopardize the blood drainage and flow to the brain. A high ICP may also result in the “herniation” of brain tissue from one compartment into another, which is a potentially fatal consequence that further inhibits CSF flow and feeds the cycle of increasing intracranial pressure. Ultimately the content of the cranium is displaced downwards due to the high ICP. It is noteworthy that the CSF develops more slowly when the ICP is elevated.
All these accommodative volume-pressure relationships occur concurrently and are subsumed under the term intracranial elastance (Figure 1)1.
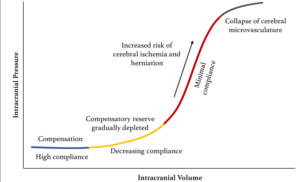
Figure 1: Pressure-volume curve for ICP. The pressure-volume curve has four ‘zones’: (1) baseline intracranial volume with good compensatory reserve and high compliance (blue); (2) gradual depletion of compensatory reserve as intracranial volume increases (yellow); (3) poor compensatory reserve and increased risk of cerebral ischemia and herniation (red); and (4) critically high ICP causing the collapse of cerebral microvasculature and disturbed cerebrovascular reactivity (grey).
As the volume of brain, blood, or CSF continues to increase, the accommodative mechanisms fail and ICP rises exponentially, in an idealized elastance (compliance) curve. At an ICP of about 25 mm Hg, the normal curve’s form starts to ascend steeply. After this point, small increases in intracranial volume result in marked elevations in ICP. This is particularly important because in clinical practice an ICP below 15 or 20 mm Hg is often given as a therapeutic target because the elastance curve becomes steep beyond this level and small increments in cerebral volume may cause large elevations in ICP.
Accordingly, a raised intracranial pressure is a medical emergency because it might jeopardize cerebral perfusion and damage the neuronal tissue by leading to ischemia and infarction. The typical clinical manifestations of an increased ICP are drowsiness, nausea, headache, ataxia, vomiting, confusion, ocular palsies, and papilledema which is the optic disk swelling. It is usually present if the raised pressure has been longstanding, but because it takes time to develop it may be absent on physical examination. It is a medical emergency; as permanent vision loss can ensue. Visual changes can range from blurred vision, double vision from cranial nerve defects and palsies, photophobia, and ultimately optic atrophy. Hypertension, bradycardia, and irregular respiration are clinical signs that are known as the Cushing triad and reflect an impending brain herniation.
In infants and small children, whose skull sutures have not yet closed, the clinical signs of an increased ICP take on special features: the clinical presentation may be a rapidly enlarging head size, bulging eyes, and a minimal neurological compromise. Nowadays, monitoring ICP has permitted approximate correlations to be made between the clinical signs and the levels of ICP. However, there are conditions in which the dissociation between ICP and clinical signs is striking,g and some of these signs might not bear a strict relation with the level of ICP. For instance, a mass that is contiguous to the third cranial nerve might compress this nerve at a time when ICP is little raised, and a slowly growing mass lesion might raise ICP gradually and allow for compensation to occur and cause few clinical signs.2
ICP is an essential parameter that must be within a narrow range and be able to measure it. Nils Lundberg reported on the earliest clinical studies conducted in this field. He is considered a pioneer in the direct cannulation of the ventricular system and has been credited with recording and analyzing intraventricular pressures over long periods in volunteers with a multiplicity of intracranial pathologies. He discovered that ICP is subject to periodic spontaneous fluctuations and that the cardiac and respiratory activities create a pulsatile component via cyclical changes in cerebral blood volume. He discussed three different pressure wave types, denoted by the letters A, B, and C.
Lundberg A waves (Plateau waves) are ICP increases that have amplitudes of 50– 100 mmHg and last on average for 5 to 15 minutes. They always reflect underlying pathological conditions in which intracranial compliance is markedly reduced and should alert for an impending herniation if the ICP is left untreated. B waves are ballistic waveforms that follow the blood pressure wave and are the result of blood entering the basal cerebral vessels but still have an unclear value in clinical practice. They might reflect vasomotor changes that are associated with an unstable ICP. C waves follow the respiratory cycle and are a complex result of several transmitted pressures from the thorax to the spinal fluid compartment, so they reflect normal interactions between the cardiac and pulmonary cycles. They have been documented in healthy adults and have no clinical significance. (figure 2)3
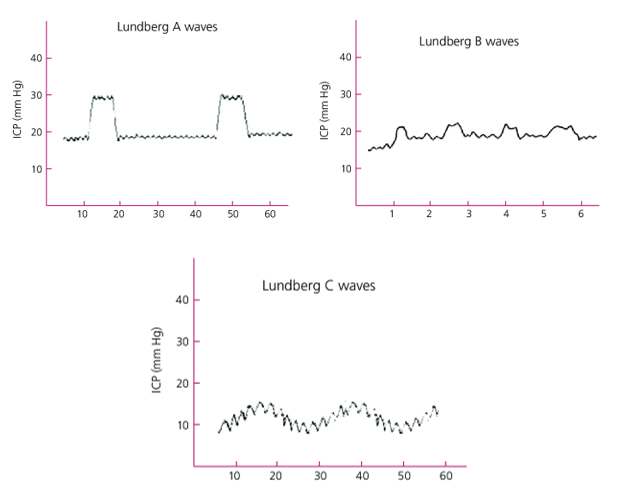
Figure 2: Lundberg waves. Lundberg A waves are ICP rises for 5 to 10 minutes, indicating upcoming herniation. Lundberg B waves have clouded value clinically. Lundberg C waves are related to the normal interactions between the cardiac and pulmonary cycles. 62
Indications of intracranial pressure monitoring
ICP monitoring has been investigated in many ways as a diagnostic and treatment approach for a variety of clinical disorders that culminate in neurological damage. ICP monitoring is helpful—if not necessary—in cases of head injury,poor-gradee subarachnoid hemorrhage, stroke, intracerebral hemorrhage, meningitis, acute liver failure, hydrocephalus, benign intracranial hypertension, craniosynostosis, etc. CSF dynamic testing in hydrocephalus helps with diagnosis and subsequent shunt function monitoring.4
Traumatic brain injury (TBI) is the most common cause of ICP monitoring (TBI).5 Clear recommendations for ICP monitoring in TBI are provided by the American Brain Trauma Foundation (BTF) standards. ICP monitoring is suggested in patients with severe TBI and a normal CT scan if two or more of the following characteristics are present at admission: Older than 40 years; Systolic blood pressure less than 90 mmHg.6 The foundation recommends (Level II B) the use of ICP monitoring in patients with TBI to decrease in-hospital and two-week post-injury mortality 58 based on the quality of the existing data. It’s important to note that the lower limit for intracranial hypertension in the current BTF guidelines is 22 mm Hg, whereas it was 20 mm Hg in the previous one.58 Additionally, it’s essential to consider the most practical site to place the ICP monitor and the effects of regional intracranial pressure gradients when ICP monitoring is being done. However, a recent multi-centric controlled trial (BEST TRIP trial) found that for patients with severe TBI, Imaging- and clinical-based care were not superior to treatment aiming at maintaining ICP at 20 mm Hg or less.7
Invasive Methods in ICP Monitoring
The preferred and suggested procedures include an intraparenchymal strain gauge or fiber optic monitor and an intraventricular monitor utilizing a ventriculostomy (EVD).8
Several different invasive methods of measuring ICP exist. Depending on the technique, ICP measuring can be undertaken in different intracranial anatomical locations: intraventricular, intraparenchymal, epidural, subdural, and subarachnoid. (figure 3)9
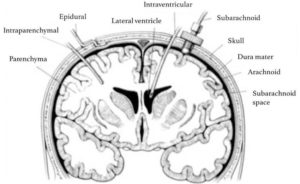
Figure 3: Sites for invasive ICP monitoring. These sites represent actual and potential spaces in the intracranial cavity in which ICP can be measured. Intraventricular monitoring with EVDs is the most commonly accessed site in clinical practice, followed by intraparenchymal probes.
External Ventricular Drainage (EVD)
The gold standard of ICP monitoring is invasive monitoring using the EVD technique, in which a catheter is inserted into one of the lateral ventricles through a burr hole. This approach is the standard against which more recent monitors are calibrated. 10, 11, 12, 13 It entails attaching a fluid-coupled external strain gauge to the catheter in one of the ventricles. An advantage of such an assembly is recalibration. Although the Foramen of Monroe is the ideal reference point for this calibration, it is typically more convenient to use the external auditory meatus. With each change in head position, the EVD requires adjusting the transducer level. (figure 4)14
The most accurate method in the current use is direct intraventricular ICP monitoring, which also has the benefit of being relatively inexpensive.
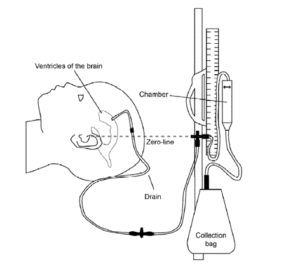
Figure 4: An external ventricular drain (EVD) is a temporary method that uses gravity to drain cerebrospinal fluid (CSF) out of compartments in the brain, called ventricles, via a thin tube that goes out of the head into a chamber and bag. The tube is inserted into the brain while your child is under a general anesthetic in the operating theatre. The tube is stitched in and the insertion site is covered with a dressing.
In addition to monitoring ICP, the placement of an EVD has other benefits such as aiding therapeutic CSF drainage, intrathecal drug administration such as antibiotics in the case of ventriculitis, or the injection of thrombolytics in the event of intraventricular hemorrhage or clotting at the proximal catheter. Additionally, the implantation of an EVD may be necessary to drain posttraumatic hemorrhage.
However, this method offers a unique set of drawbacks. Even though it seems like a routine surgical procedure, hemorrhage, and infection complications are frequent. While there are varyingly high rates of hemorrhage in different case series, hemorrhage was more frequently detected in hospitals that routinely performed CT scans following the procedure.15 Another study 16 with a total of 188 EVD patients reported “postoperative hemorrhages” in 41% of cases, after being scanned by CT postoperatively, however only 10.6% had hemorrhages larger than 15 mL or with an intraventricular component.
Bacterial colonization of the catheter and consequent retrograde infection are other complications when dealing with EVD. This covers a broad range of illnesses, from benign skin infections to meningitis, ventriculitis, and deadly septicemia.
It is the most invasive of all available techniques, as the ventriculostomy penetrates the meninges and brain, with the risk of bacterial transmission through fluid coupling.17, 18 The documented risk of infection varies between <1% and 27% in various studies, the mean EVD‑associated infection rate is 8–9%.
Numerous techniques have been shown to lower infection rates, including the use of antibiotic- and silver-impregnated catheters, subcutaneous placement of catheters, minimal mechanical disruption of the EVD setup, limiting the duration of EVD to less than five days, and the use of sterile techniques in an operating room setting 17, 19, 20, 21 Given that there is currently insufficient data to support either prophylactic antibiotics or routine catheter changes, it would be advisable to avoid routine CSF sampling. 18, 19
Another disadvantage of the intraventricular approach is that it can be difficult to introduce an EVD in young patients whose ventricles are narrowed or slit-like due to cerebral edema.22 In these situations, the ICP waveform may be dropped and the recorded values may be low due to the artifact effect.
The ipsilateral lateral ventricle anterior to the foramen of Monro or the top of the third ventricle are the best locations for EVD. However, the choroid plexus near the bottom of the third ventricle should be avoided. The preferred site is the right hemisphere, which is non-dominant in 90% of the population. Many retrospective studies have detected misplacements, either intraparenchymal or extraventricular.23 When ventricular cannulation is attempted improperly or enthusiastically, the basal ganglia may be damaged. The catheter should be carefully examined for kinks, and the drainage tube should be checked for air bubbles, blood clots, or debris that could obstruct the free passage of CSF or result in a dampened waveform.24,25
Subarachnoid devices
Another fluid-coupled device, this one uses saline-filled tubing to connect the intracranial space to an external transducer at the patient’s bedside. The burr hole used to insert the subarachnoid bolt serves as a hollow screw. A spinal needle is used to puncture the dura at the base of the bolt, allowing the subarachnoid CSF to fill the bolt.26 After that, pressure tubing is linked to communicate with a pressure monitoring system. It is no longer typical practice to monitor ICP using this technique. The benefits include little invasiveness and a reduced risk of infection. However, they are prone to errors such as ICP underestimation, screw positioning problems, and obstruction by debris.27
Epidural/subdural devices
They are an attractive choice because they are the least invasive and can be installed fast and easily. However, compared to intraventricular or intraparenchymal devices, they are less preferred options because of their doubtful accuracy and reliability as well as a baseline drift, which after more than a few days of use, can surpass 5 ± 10mmHg.28
Other invasive methods
In the current era of neurosciences, another method to directly monitor ICP is the use of enormous advancements in micro-transducer technology. These ICP micro-transducers can be used at any location – intraventricular, epidural, subdural, or intraparenchymal. The right frontal cortex parenchyma is the most typical location for micro-transducer installation in routine practice, while other sites may also be chosen in the brain parenchyma based on loco-regional pathology.29
Micro-transducers and intraventricular devices have shown a strong association. They have an advantage over EVD in terms of ease of use and the fact that readings are unaffected by patient postures. The lack of a fluid-coupled system reduces the risk of infection, and the ease of the placement of surgical maneuvers results in fewer hemorrhagic consequences. Additionally, compared to fluid-coupled devices like the EVD, the incidence of pressure waveform damping and measurement artifacts is lower.29,30
The disadvantages of intraparenchymal transducer technology include the inability to recalibrate, therapeutic CSF drainage is not possible, inability to predict global ICP in addition to the drift in the system that develops with continued use.30
Fiberoptic catheter tip transducers (Camino intracranial pressure monitor)
This comprises a fiberoptic transducer that calculates ICP and represents it as both a waveform and a number by detecting changes in light signal intensity using a diaphragm and microprocessor-driven amplifier.31 Typically, a daily baseline drift of 0.3 mm Hg takes place.31, 32, 33 Available research indicates that the substantial infectious and hemorrhagic signs are negligible.32, 33, 34 Ineffective fiberoptic cables have been primarily attributable to technical errors.31, 32
Strain Gauge Micro-Transducer ICP Monitoring Devices
Diffuse piezoelectric strain gauges are located on a silicon microchip at the transducer tip. The microsensor measures ICP at the intraparenchymal, intraventricular, or subdural source, and transmits the data electronically rather than through a hydrostatic system or fiberoptics. Extensive research has proven the Codman microsensor to be a reliable, stable, and accurate ICP monitoring system that, when used in conjunction with the ventricular catheter, provides therapeutic alternatives for CSF drainage.30 Due to its small size, it can be used at various anatomical sites and in the pediatric age range. Alignment and zeroing are unnecessary, and most critically, infection and hemorrhagic consequences are minimal.35, 36, 37
Pneumatic Sensor (Spiegelberg Brain Pressure Monitor)
These ICP monitors primarily measure ICP at subdural or epidural locations38 by using pneumatic strain gauze technology in a balloon-tipped catheter device. With minimal infections and hemorrhagic consequences, the available evidence has demonstrated results that are comparable to the gold standard intraventricular and intraparenchymal EVD-based monitors.38, 39, 40 In addition to being less expensive than micro transducers, recent versions of these compliance monitors also feature facilities for periodic automatic drift correction, which has made them quite popular in neurocritical care setups.30
Non-Invasive Methods in ICP Monitoring
An invasive intracranial ICP monitor used in a direct measuring strategy is the most reliable way to properly diagnose increased ICP. However, invasive monitoring may not always be used due to the invasiveness of these monitoring techniques (which request inserting an ICP sensor into the ventricles or brain parenchyma), additional risks to the patient may present such as hemorrhage and infection, high costs associated with ICP sensor implantation, and limited access to trained personnel, i.e., a neurosurgeon/neuro-intensivist in suburban areas. Alternative methods to assess ICP non‑invasively have, therefore been sought. All ICP monitoring procedures are based on a thorough clinical evaluation together with imaging modalities (CT head and MRI).
Computerized Tomography
Several attempts have been made to develop ICP and mortality prediction models based on CT abnormalities such as loss of gray and white matter distinction, midline shift, and basal cistern and ventricular effacement.41, 42, 3 Current data, however, suggests that CT is not a very sensitive instrument because CT remains normal even when ICP is elevated.44
Magnetic Resonance Imaging
The MRI-ICP method is a novel approach that predicts ICP by combining advancements in MRI technology with neurophysiological principles.45 To estimate the mean ICP, it essentially measures the pulsatile variations in intracranial volume and pressure.
Additionally, MRI has been utilized to assess the optic nerve and estimate the diameter of the optic nerve sheath 3 mm posterior to the globe.46 However, MRI-based approaches have an obvious gap in that they only provide an image of ICP at a specific moment. Additionally, bedside operations are not convenient when using MRI-based techniques, which subject the patient to the logistics of transportation.47
Transcranial Doppler Ultrasonography
Using ultrasound, this method measures the blood flow velocity through the main intracranial vessels, most frequently the middle cerebral artery. Transcranial Doppler (TCD) tests can estimate elevated ICP since it interferes with CBF and lowers blood flow velocity as a result. In addition to the mean velocity, the slopes of the TCD waveforms and the pulsatility index have been linked to ICP. The pulsatility index is calculated as the difference between the peak systolic and end diastolic velocity, divided by mean flow velocity.48–50 However, there is a 10 to 15 mm Hg range of error in the estimates.51 Additionally, the approach needs practice, and variances between and within observers are possible.52 Additionally, it could be challenging for the 10-15% of patients who don’t have a sufficient bone window.
Tympanic Membrane Displacement
The method takes advantage of the perilymphatic duct’s role as a conduit for CSF and perilymph communication. Tympanic membrane movement brought on by stimulation of the stapedial reflex has been correlated to ICP.53, 54 Stapes are perched on the membrane-covered oval window. This membrane is flexible, which means that its location and movement are influenced by the pressure of the fluid within the cochlea. The core of this method is a quantitative measurement of this movement. According to available research, tympanic membrane infrasonic emissions and TMD are reliable screening tools that can be used to evaluate and monitor individuals with elevated ICP. They do not, however, provide the measurement of precise ICP levels, and the test has some requirements, including intact stapedial reflexes, normal middle ear pressure, and a patent cochlear aqueduct.54–57
Optic Nerve Sheath Diameter (ONSD)
Since the optic nerve is a component of the central nervous system, the dural sheath surrounds it. A tiny 0,1-0,2 mm subarachnoid space exists between the sheath and the white matter and connects to the subarachnoid space enclosing the brain. The sheath enlarges when ICP is elevated. Using transocular ultrasound, the diameter of the nerve sheath can be seen to change (Figure 5).58 Numerous research 46, 59–61 have looked into the relationship between invasively determined ICP and nerve sheath diameter. It was discovered that the correlation coefficient ranged from 0,59 to 0,73. This method is inexpensive and effective; each patient’s evaluation lasts around 5 minutes.59 In multiple investigations, it has been proven to be a reliable screening technique for identifying patients with elevated ICP who require treatment and whose optic nerve sheath diameter (ONSD) is greater> 5 mm, which corresponds to an ICP of 20 mm Hg or above.60,61 Sonographic ONSD assessment has certain restrictions even though it’s uncomplicated. It is not recommended for patients with lesions that are clinically frequent, such as tumors of the orbit, ocular inflammation, Graves’ disease, sarcoidosis, disorders that alter the optic nerve sheath diameter, and people with optic nerve lesions.29
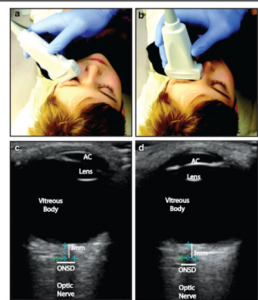
Figure 5: The evolution of ultrasound technology and the development of high-frequency (> 7.5 MHz) linear probes with improved spatial resolution has enabled excellent views of the optic nerve sheath.
In situations where invasive ICP monitoring techniques are either not practical (patients with severe coagulopathy) or not available, ONSD is a potential technique for screening patients with high ICP, setups without access to a neurosurgeon.
Conclusion
The article exposed and demonstrated the importance of using ICP monitoring systems and their indications in clinical practice and why it is important to keep monitoring this parameter in patients with high ICP to confirm and initiate ICP lowering measures as soon as possible and to assess if these interventions are effective or to avoid unnecessary treatment in patients with normal ICP. These monitoring systems are especially important when the patients are sedated and paralyzed and neurological assessment and examination cannot be performed by the clinician. There also had been evidence, mainly from retrospective cohorts of patients with brain injury, that the outcome in patients was better if the ICP was maintained at adequate levels well below those that compromise cerebral perfusion which can be precisely achieved by using the ICP monitoring systems.
References